Quantum
computing
Building the computer of the future
Quantum computers have the potential to perform extremely complex calculations, by encoding information into quantum states. This opens the way for revolutionary applications, such as complex optimization challenges or prediction, simulation, and modelling of the behaviour of molecules, catalysts, and new materials.
Realizing the promise of quantum computing requires the development of different layers of hardware and software. Together, these layers are referred to as the quantum computing stack. This stack is what we explore at QuTech. The base of the stack – the ‘quantum processor’ – contains the qubits. We are investigating different types of qubits, along with the system architecture that translates quantum algorithms into electronic signals that operate on the qubits.
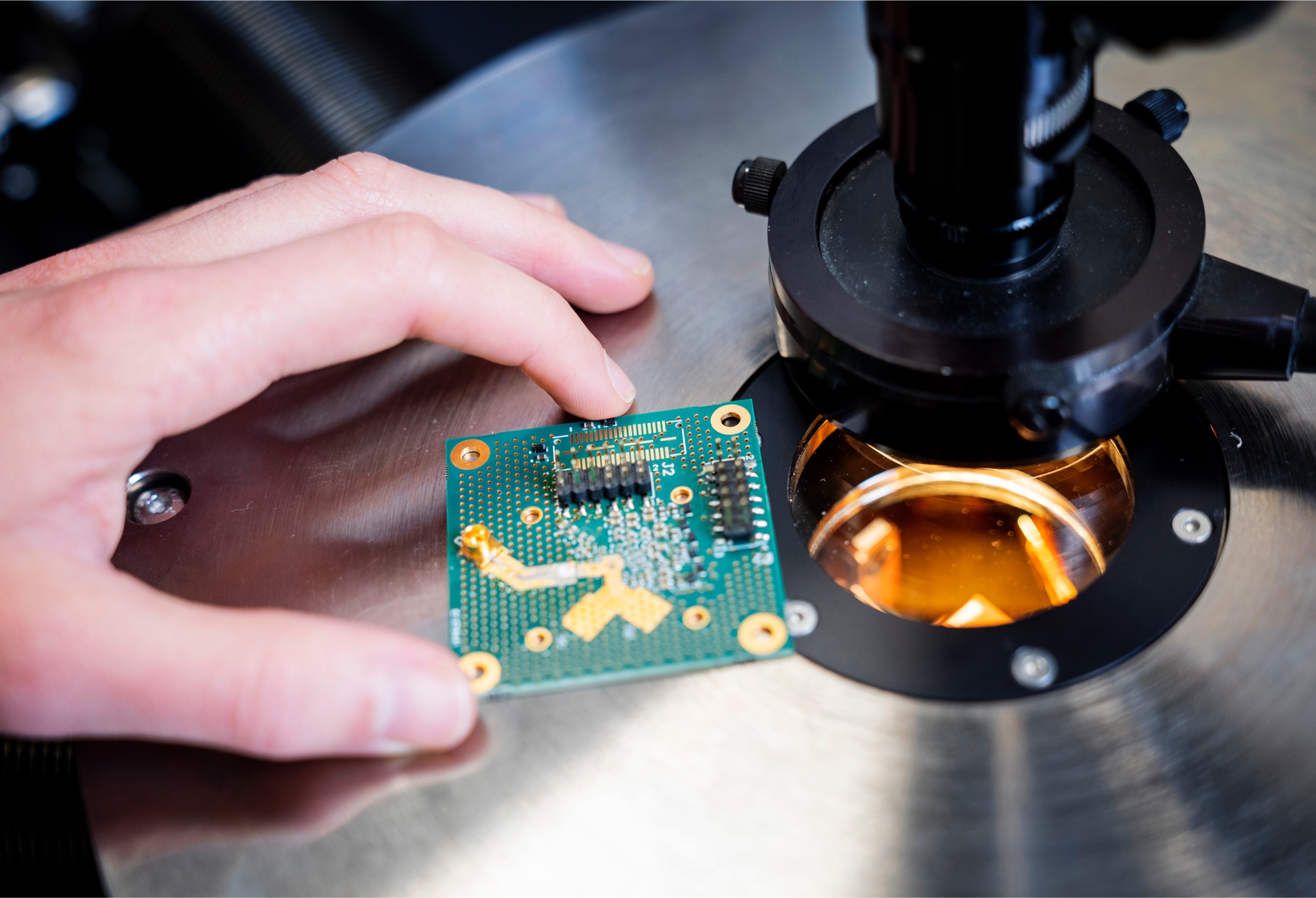
Spin qubits
Long-term goal:
To build a modular spin-qubit processor for scalable quantum information processing.
Highlights
We advanced 28Si/SiGe and Ge/SiGe heterostructures for the next generation of spin-qubit processors. In silicon we obtained two-dimensional electron gas with enhanced and more uniform transport properties across a 100 mm wafer (Appl. Phys. Lett.). We also elucidated and statistically predicted the valley splitting by the holistic integration of 3D atomic-level properties, theory and transport (Nat. Comm.). In germanium we obtained mobilities in excess of one million cm2/Vs (Appl. Phys. Lett.,). We also pioneered germanium bilayers towards 3D quantum devices (Adv. Quantum Tech., front cover).
In germanium, we programmed the first rudimentary error correction circuit by realizing the phase-flip code on a four-qubit germanium quantum processor (NPJ Quantum Information).
We reported a two-qubit gate fidelity between two electron spins in Si/SiGe quantum dots of 99.65% (Nature). This constitutes a much-anticipated achievement that brings the spin qubit field in a position to achieve fault-tolerance.
We realized a linear array of 6 electron spin qubits in a Si/SiGe quantum dot array (Nature). This experiment not only pushes the envelope of the number of qubits in silicon, it also achieves high initialization fidelities, high readout fidelities, high single-qubit gate fidelities, and high two-qubit state fidelities - all at the same time.
Working closely together with engineers from Intel, we measured the first qubits made in the very same industrial manufacturing facilities that are used to mass-produce conventional computer chips (Nature Electronics). This advance has been a long-standing goal due to its promise of scalability and was featured on the cover of Nature Electronics.
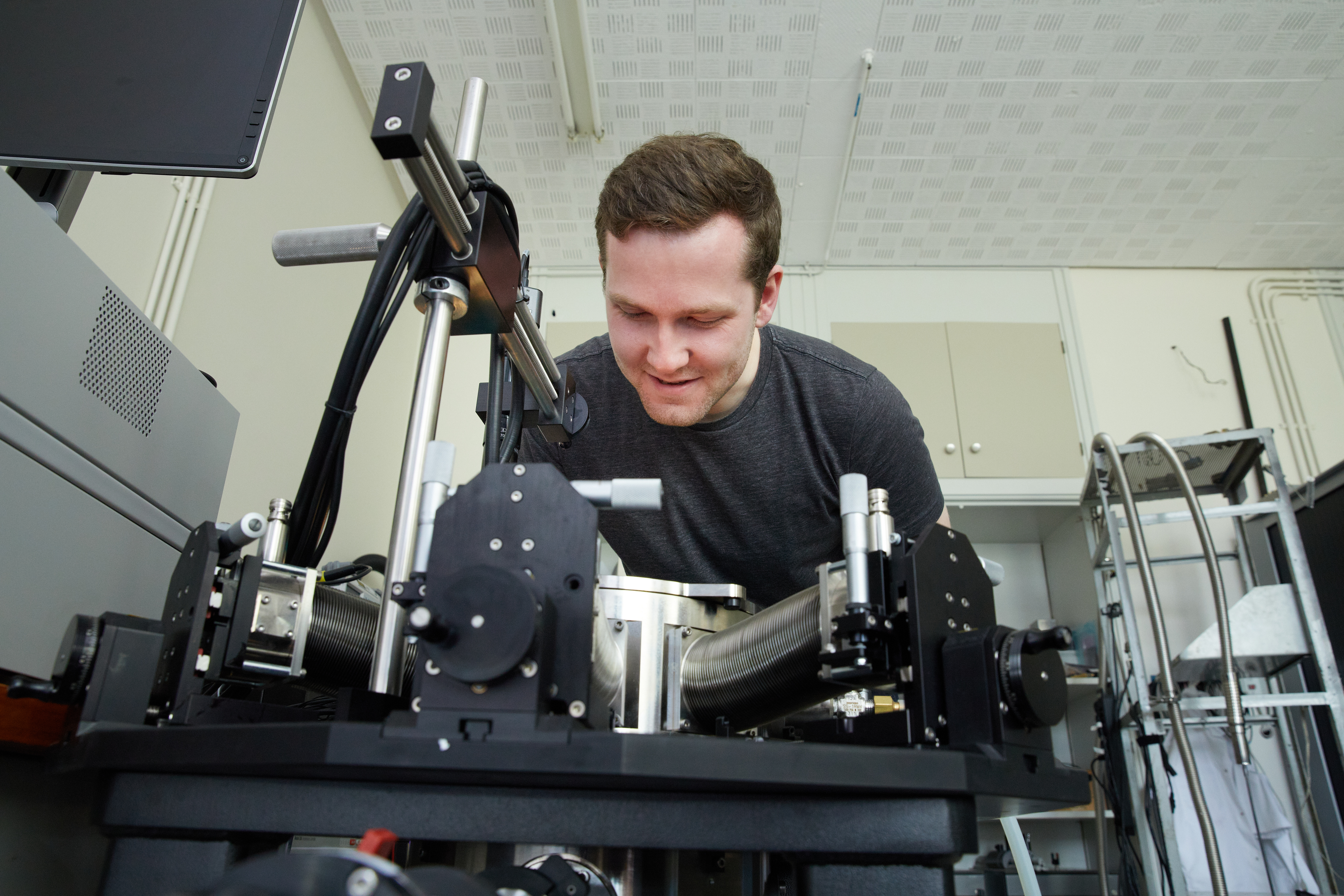
Transmon qubits
Long-term goal:
To realize an error-protected logical qubit with a superconducting circuit and a flexible control stack that also enables NISQ applications.
Highlights
Our Starmon-5 Quantum Computer available online via Quantum Inspire has undergone key upgrades, including faster two-qubit gates (from 60 to 40 ns), faster qubit readout (from 3 to 1 ms), and most importantly the ability to perform multiple measurements within a circuit, thus enabling mid-circuit measurement, as required in quantum teleportation and quantum error correction.
We joined the OpenSuperQPlus consortium of the European Quantum Technology Flagship, starting in March 2023, to build a 100-qubit computer available in the cloud within 3.5 years, in collaboration with TNO, Orange Quantum Systems, Qblox, QuantWare, and Delft Circuits.
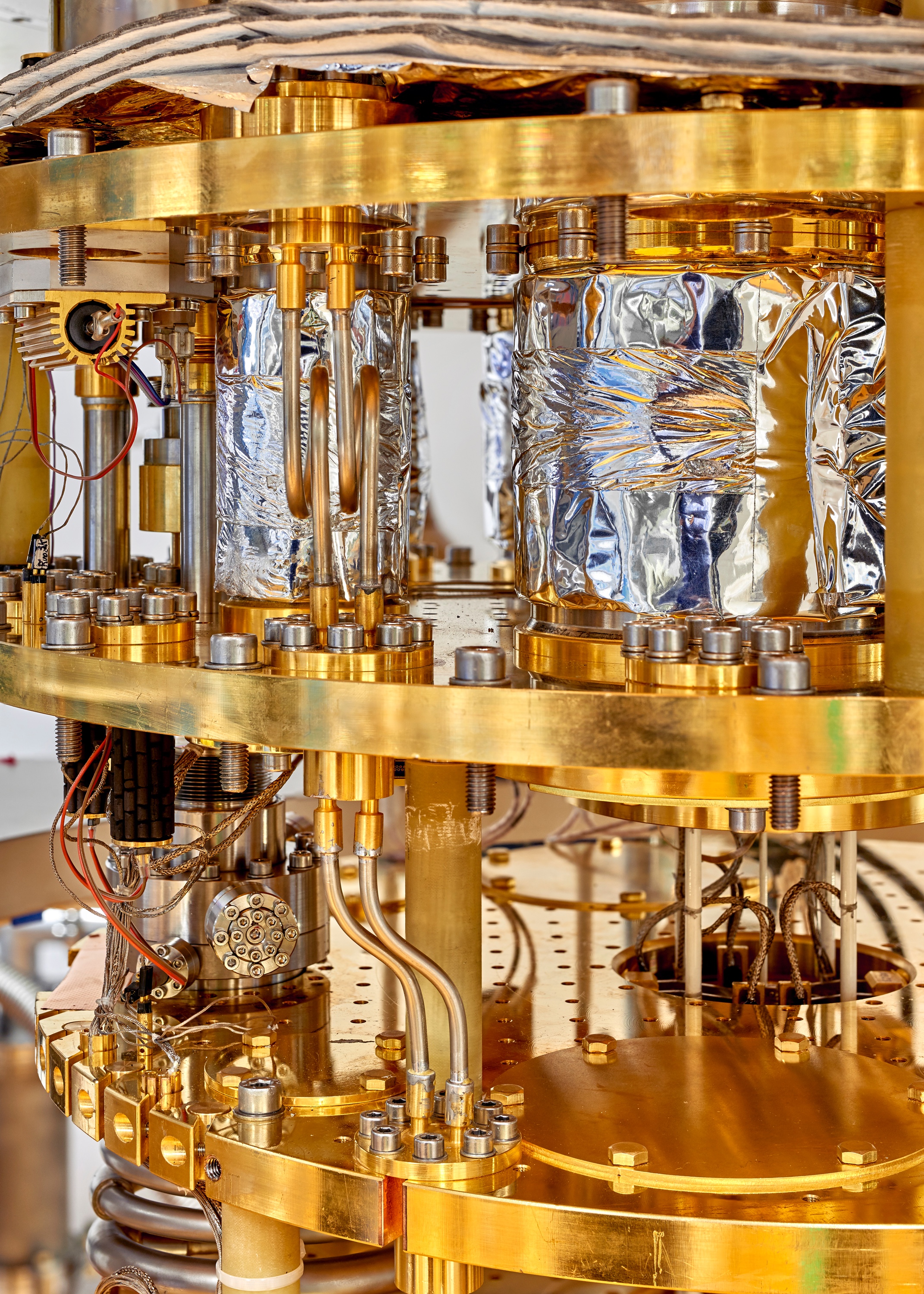
Cryogenic electronics
Long-term goal:
To replace room-temperature electronics that control quantum processors with integrated cryogenic electronics, operating in close proximity to the qubits, in order to facilitate large-scale quantum computers.
Highlights
We demonstrated a cryo-CMOS digital-to-analog converter for multiplexed spin-qubit biasing, able to bias up to 5000 spin qubits while keeping an extremely low power consumption compatible with sub-K refrigeration stages (VLSI Techn. and Circuits).
To provide the frequency reference for the qubit control and readout circuits, we developed a calibration technique to minimize the phase noise of cryo-CMOS voltage-controlled oscillators (TCAS-I), and we demonstrated the first cryogenic phase-locked loop (PLL) operating at 4.2 K, able to synthesize 9.4 to 11.6 GHz tones while maintaining high qubit fidelity (JSSC).
We analysed the trade-off in the development of real-time hardware neural-network decoders for surface-code quantum-error-correction schemes, laying the basis for cryo-CMOS implementation of quantum error correction decoders. (TQE).
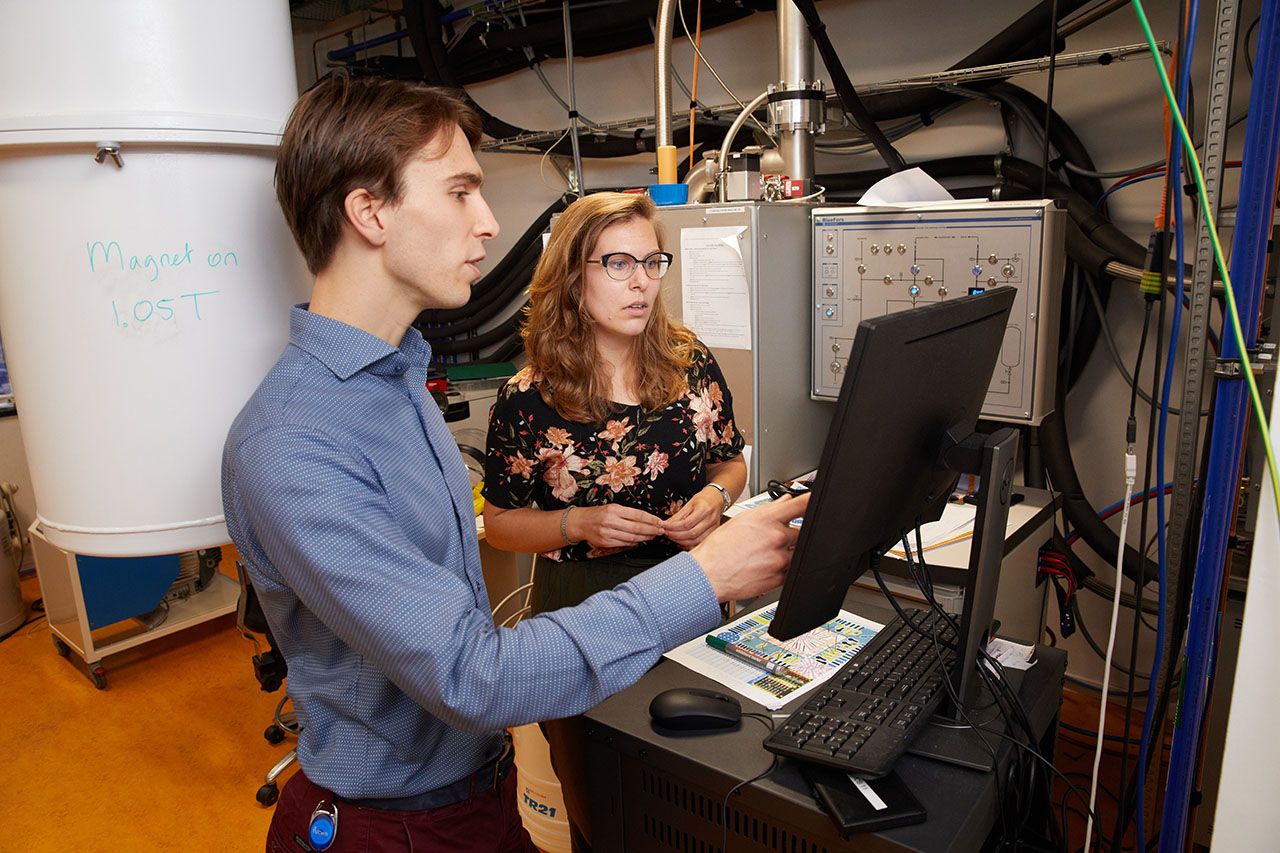
Quantum computing architecture stack
Long-term goals:
To develop a scalable quantum computing control system stack that bridges the gap between quantum applications and quantum devices.
Highlights
We investigated the need for tight co-design of different layers of a full-stack quantum computing system and emphasized the development of hardware- and algorithm-driven compilation techniques (DATE).
We continued our efforts towards modular quantum computer architectures by investigating the mapping of quantum algorithms to multi-chip quantum computers as well as characterizing the spatio-temporal traffic overhead between different chips (NANOCOM).
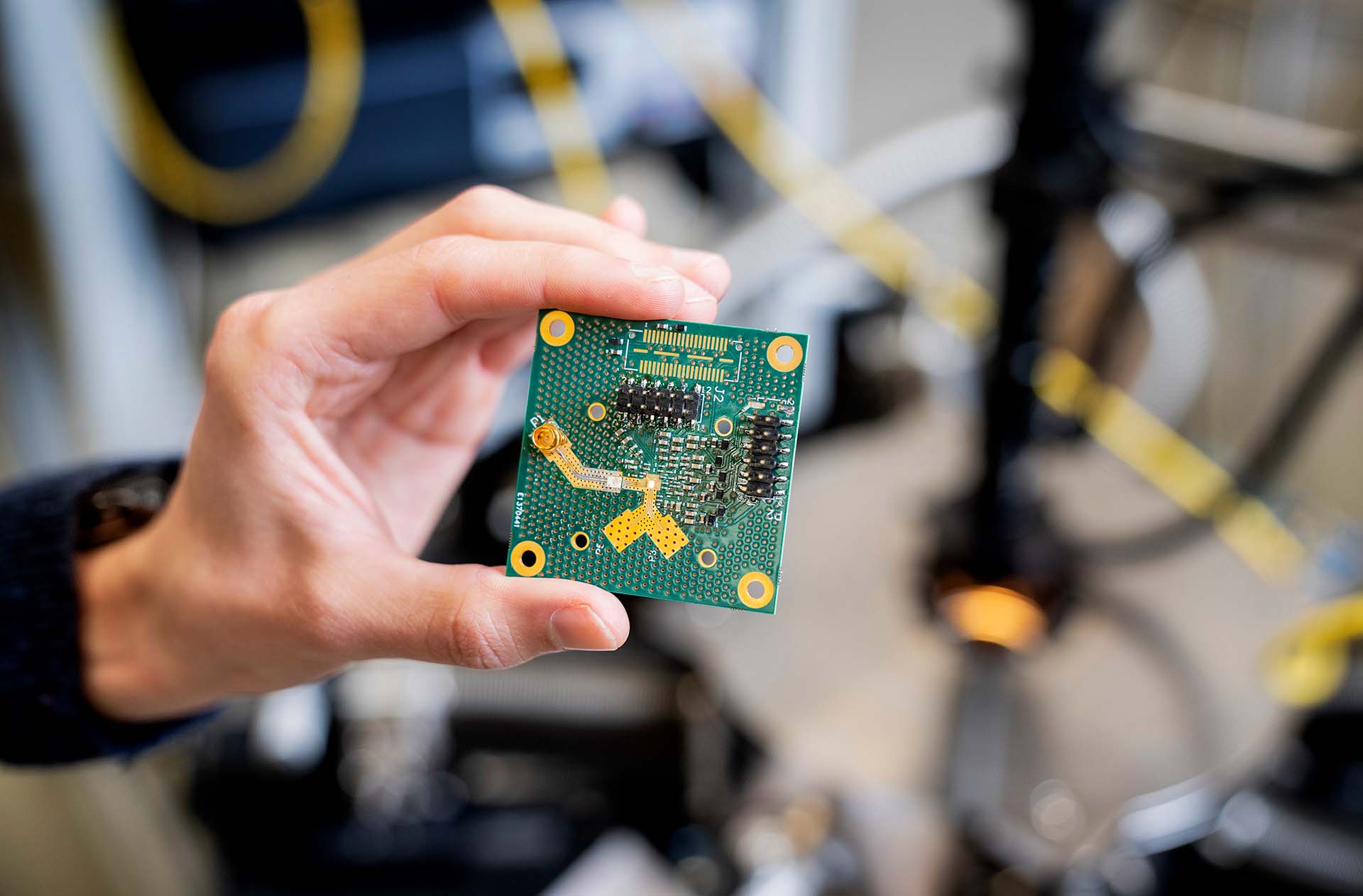
Theory for assessing the performance of quantum processors employing superconducting and spin qubits
Long-term goal:
To provide analyses and ideas towards implementing and assessing the performance of quantum error correction for superconducting and spin qubits.
Highlights
We proposed a two-qubit gate between a fluxonium and a transmon qubit for which we show high fidelity by numerical simulation, and which could function as an essential component in a hybrid transmon-fluxonium qubit code architecture. We show how the gate would avoid the problem of frequency collision in all-transmon, all-microwave architectures (Phys. Rev. Research 2022).
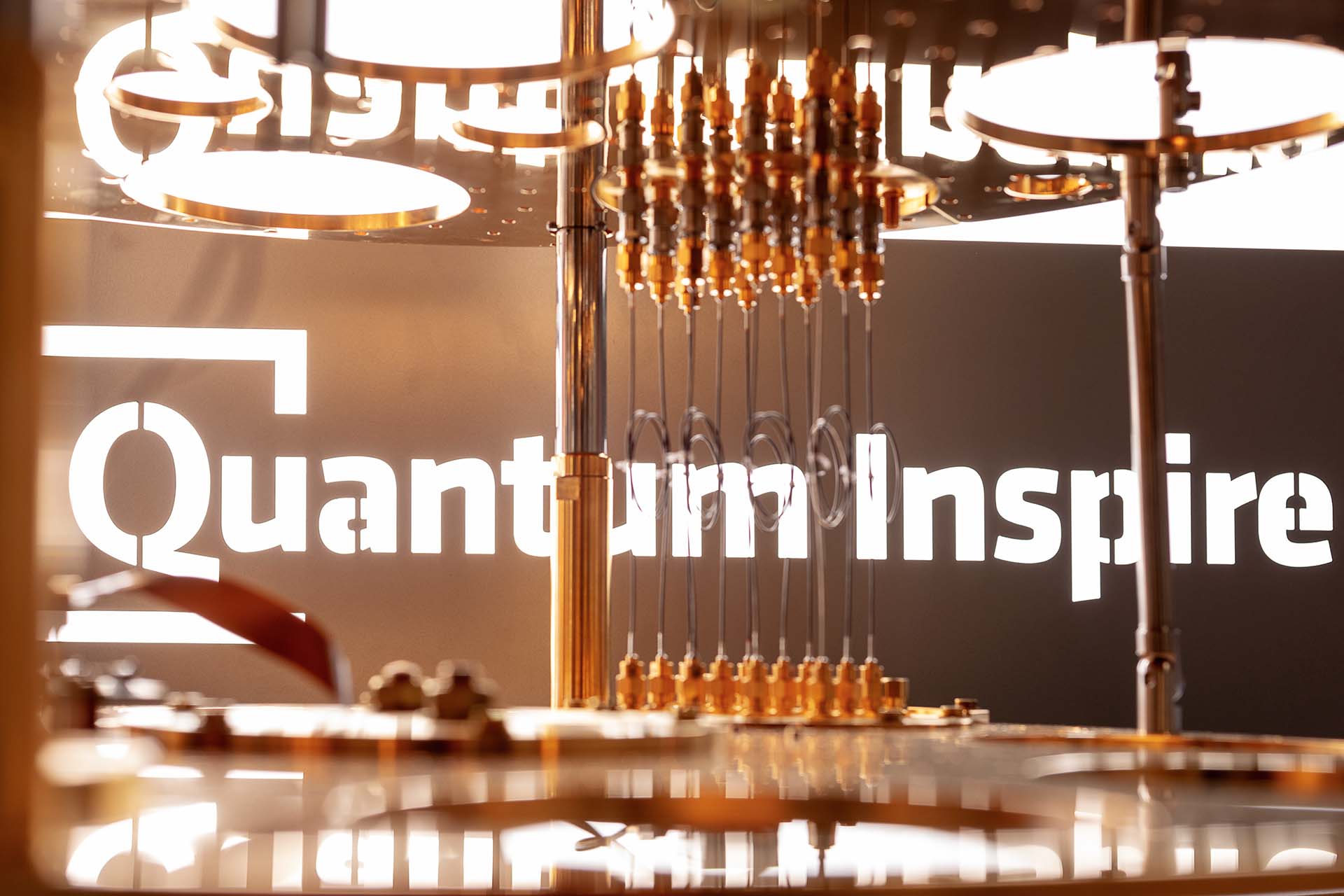
Quantum Inspire: the multi hardware quantum technology platform
Long-term goals:
To develop a full-stack quantum computer demonstrator for training and education, along with co-development and collaborative R&D of quantum technologies for quantum computing.
Highlights
We added functionality to perform mid-circuit measurements on backends that support this feature. This enables the execution of dynamic quantum circuits, where the flow is determined by the results of intermediate measurements.
We performed a huge software overhaul to support hybrid computing using both quantum resources and classical resources. This feature will become fully available in the summer of 2023 for all backends that support this feature.
We improved the duration of gates and fidelities for qubit control on the Starmon backend, increasing the length of quantum circuits that can be implemented on that hardware.
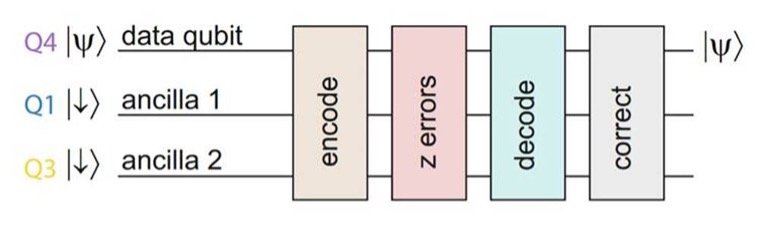
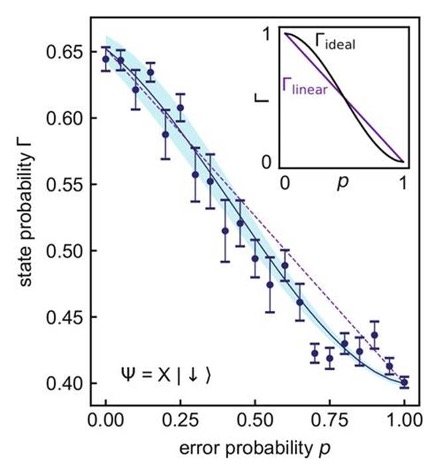
Demonstration of phase-flip error correction with semiconductor spin qubits, implemented in a 2x2 germanium qubit array. The algorithm is sketched in the cartoon and the graph shows the experimental results, demonstrating a finite region where single-qubit phase errors are corrected.
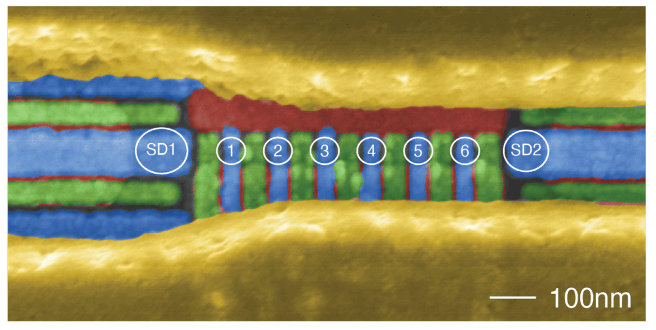
Image of the six-qubit quantum processor described in this article (Nature). The qubits are created by tuning the voltage on the red, blue, and green wires on the chip. The structures called SD1 and SD2 are extremely sensitive electric field sensors, which can even detect the charge of a single electron. These sensors together with advanced control schemes allowed the researchers to place individual electrons at the locations labeled (1)-(6), which were then operated as qubits.
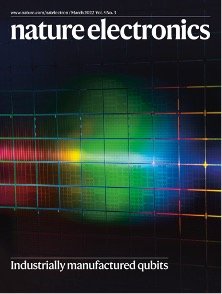