Qubit
research
Next generation of qubits for quantum computing
The number one quantum computing challenge is that qubits (the basic units in quantum computers) are extremely fragile. Qubits are easily influenced by many things, like light and temperature. Quantum decoherence is what happens as a quantum system gradually loses its special quantum behaviour over time.
To overcome this challenge, we study new types of qubits that are by design protected from outside influences. These new qubits are referred to as ‘protected qubits’ and have the potential to outperform established technologies. We aim to understand, develop, and demonstrate protected qubits. In our efforts we combine material science, quantum theory, and novel device design.
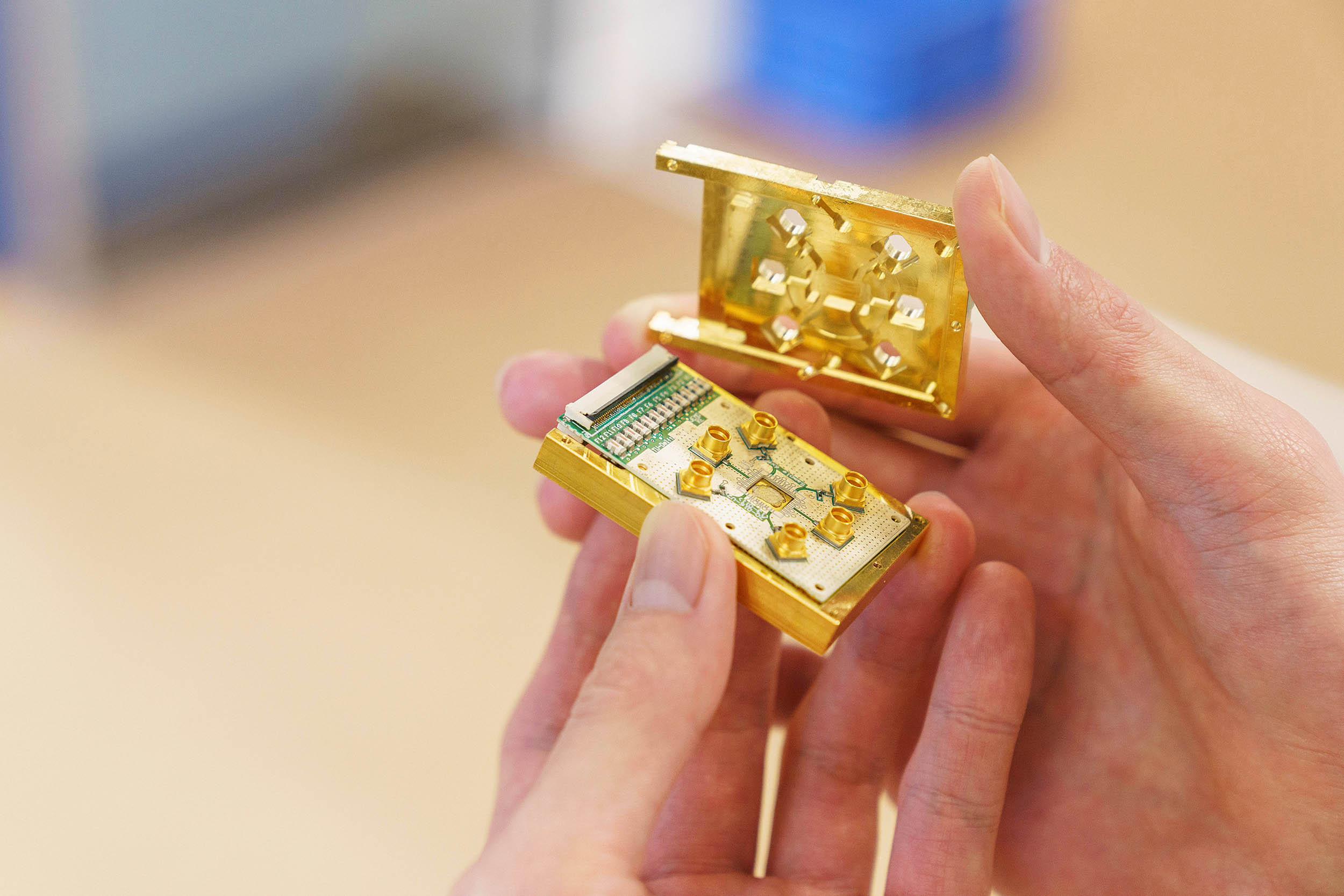
New avenues for Majorana qubits
Long-term goal:
To develop a platform for topologically protected qubits with long coherence times and fault-tolerant quantum operations.
Highlights
We used quantum dot superconductor hybrids to reliably engineer and study Majorana zero modes (MZMs) in a scalable and flexible two-dimensional platform (arXiv, Nature Comm.). We revealed the microscopic processes that govern the interactions between quantum dots (Phys. Rev. X), leading to the formation of Majoranas. We showed theoretically (arXiv) and experimentally (arXiv) how strong coupling to a superconductor gives rise to more protected MZMs. Moving towards a Majorana-based qubit we demonstrated experimental techniques for spin-filtered measurements (Nature Comm.), fast gate-sensing (arXiv), and charge-sensing (arXiv) of hybrid systems, and developed new protocols for the manipulation of MZMs (Phys. Rev. B).
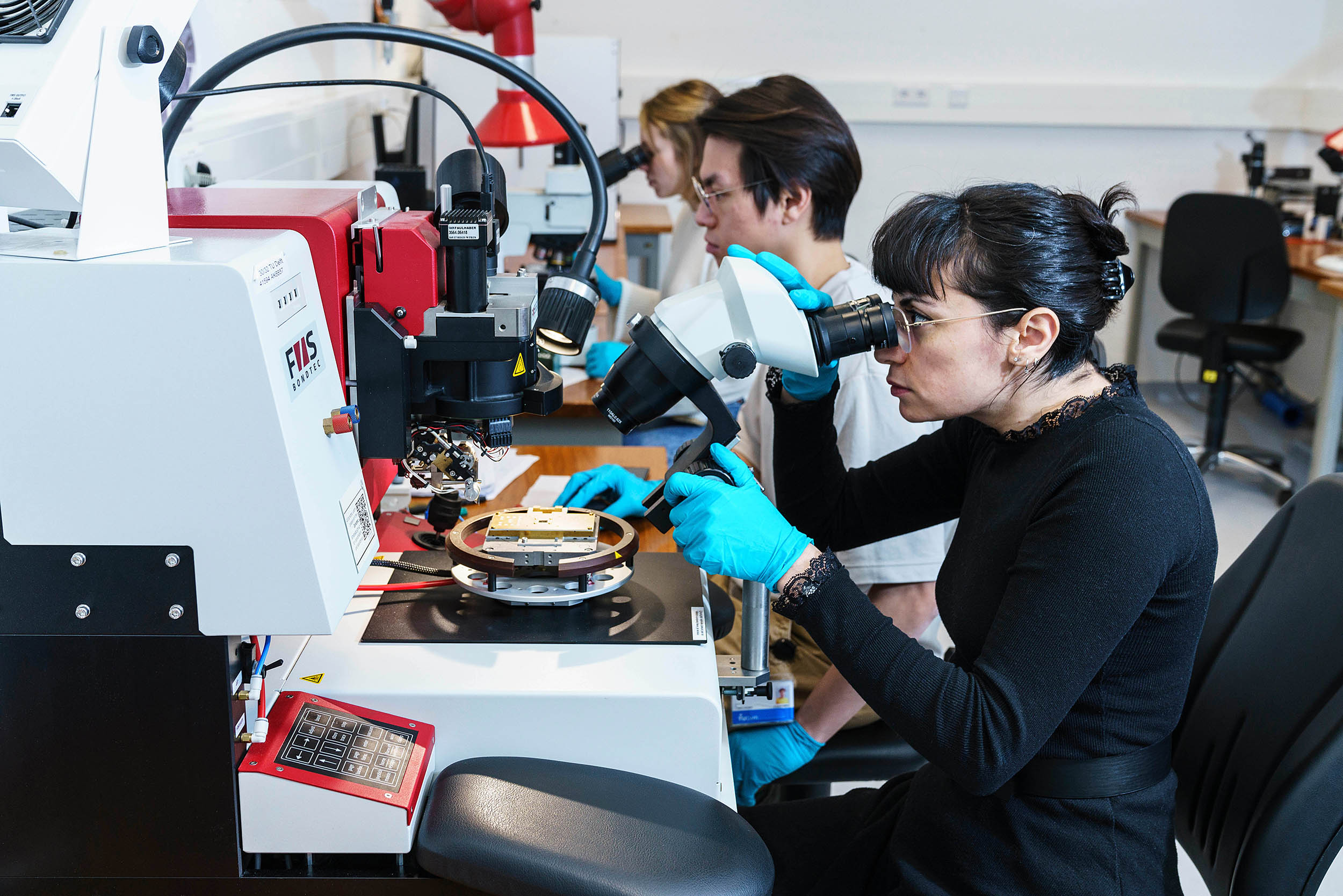
Novel superconducting and hybrid qubit architectures
Long-term goal:
To develop new qubits based on superconducting circuits with intrinsic protection against noise and with gate fidelities outperforming conventional qubit architectures.
Highlights
Using a nanowire transmon hosting a quantum dot inside its Josephson junction, we studied how the spin-orbit coupling allows for direct driving of the spin transition of the quantum dot (Phys. Rev. Lett.). Moreover, we implemented a novel superconducting spin qubit in the same platform (Nature Physics) and demonstrated tunable long-distance coupling between distant superconducting spin qubits (arXiv). We also used similar hybrid nanowires to develop a new kind of gate-tunable parametric amplifier (Phys. Rev. Applied), capable of providing on-chip amplification for cryogenic quantum circuits. Our research on fluxonium qubits has allowed us to develop a novel flux-pulse-assisted readout scheme (arXiv) that offers significant improvements in the signal-to-noise ratio for reading out protected qubits.
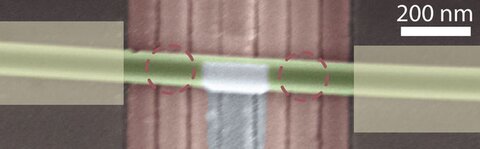
SEM image of a nanowire-based quantum dot-superconductor hybrid device to realize Kitaev chains
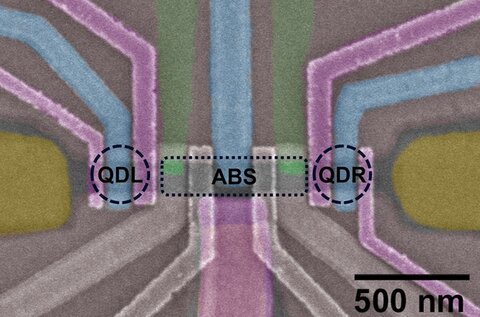
SEM image of a 2DEG-based quantum dot-superconductor hybrid device to realize Kitaev chains
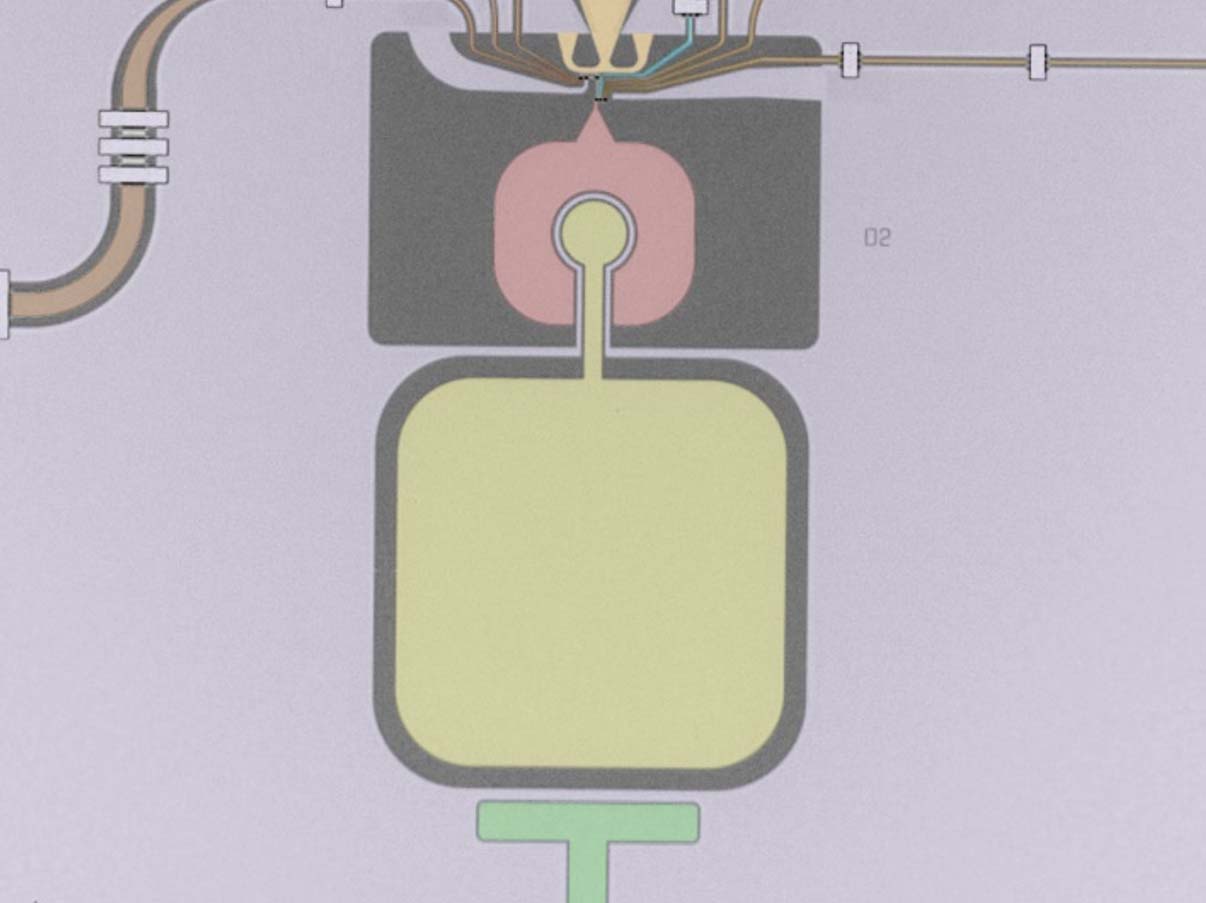
Optical micrograph of a transmon island hosting two superconducting spin qubits coupled to a superconducting resonator