Although quantum computers will not replace classical computers in the near future, they have the potential to perform calculations that need far more computing power than classical computers will ever possess. This opens the way for revolutionary applications, such as complex optimization challenges, or prediction, simulation and modelling of the behaviour of molecules, catalysts and new materials.
Realising the promise of quantum computing requires the development of different layers of hardware and software. Together, these layers are referred to as the quantum computing stack (pictured below), and this is what we explore at QuTech. The base of the stack – the ‘quantum processor’ – contains the qubits. We are investigating different types of qubits, each with its own advantages, along with the system architecture that translates quantum algorithms into electronic signals that operate on the qubits.
Spin qubits
Long-term goal:
To build a modular spin-qubit processor for scalable quantum information processing.
Highlights:
- We demonstrated state-of-the-art planar germanium for qubit arrays (Materials for Quantum Technology, Phys. Rev. B).
- We achieved an advanced understanding of valley splitting in silicon and substrates for multi-qubit experiments (Physical Review Letters).
- We demonstrated high-throughput electrical measurements of quantum materials and devices (npj Quantum Information).
- We demonstrated state-of-the-art qubit performance using silicon quantum dots made using advanced semiconductor manufacturing in the Intel industrial facilities (arXiv). This fulfilled a long-term promise of silicon-based spin qubits, and marked a major milestone in our partnership with Intel.
- We introduced and implemented a method for spin readout at a distance that uses a cascade of electrons displacing each other, analogous to toppling dominoes (Nature Comm.).
- We demonstrated, for the first time, universal quantum logic with hot silicon qubits[ST1] (Nature).
- Quantum dot arrays were fabricated in silicon, in silicon quantum wells, and in germanium quantum wells using an overlapping gate approach (Appl. Phys. Lett.).
- We demonstrated that a single hole (the absence of an electron) can be coherently controlled (Nature Communications).
- We defined a roadmap for quantum information using germanium (Nature Review Materials).
- We reported the longest spin relaxation times for holes by defining hole spin qubits in germanium (Nano Letters).
- We demonstrated the first two-dimensional quantum dot arrays using standard manufacturing materials, by realizing a gate-defined 2x2 quantum dot array in germanium (Appl. Phys. Lett.).
Qubits and control electronics operating in the same temperature regime to enable integrated quantum circuits.
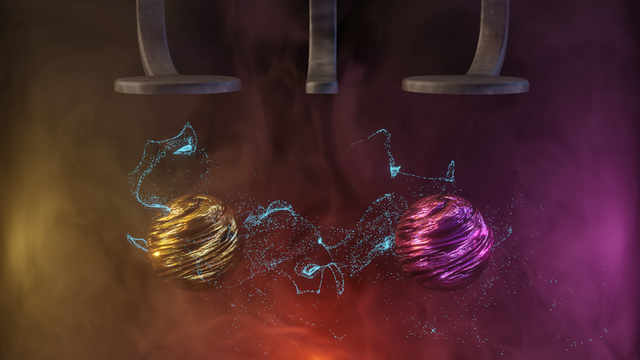
Transmon qubits
Long-term goal:
To realize an error-protected logical qubit with a superconducting circuit and a flexible control stack, enabling NISQ applications.
Highlights:
- High-fidelity two-qubit gates operating at the speed limit of superconducting quantum processors (arXiv).
- Fault-tolerant quantum operations in a surface-code logical qubit stabilized using repetitive error detection (arXiv).
- Public release of semiconducting Spin-2 and superconducting Starmon-5 quantum computers in the cloud via QuTech’s Quantum Inspire platform.
Majorana qubits
Long-term goal:
To develop robust qubits with
a long decoherence time. Our main focus is on qubits based on Majorana bound states, for which we want to demonstrate the principle of topological protection.
Highlights:
- Reading out a topological qubit can be achieved using an interference experiment, measuring the phase difference acquired by electrons passing through different paths. On the device level, this requires a closed loop of semiconductor material. Together with collaborators from Eindhoven, we were able to fabricate and measure proof-of-concept loop devices which we could use to measure phase lapses due to transmission through a quantum dot. These experiments allowed new insights into the design of future experiments with topological systems (Nature).
- Charge islands with controllable contacts to reservoirs are a necessary ingredient for topological qubits. Using a gatemon (a transmon qubit with a gate-controllable nanowire junction) we investigated the behaviour of the charge islands in the limit of a transparent, single-channel contact to an outside reservoir – where we could experimentally verify for the first time a long-standing theoretical prediction on the suppression of charging effects. Our results also suggest novel approaches for building charge-insensitive qubits (PhysRevLett.).
- Essential to the design of topological qubits are quantum dots integrated alongside the topological superconducting regions. In parallel to nanowire-based geometries, we are also working on 2D semiconductor materials. Our focus particularly lies on InSb 2D electron gases, which exhibit a large g-factor and spin-orbit interaction beneficial for topological superconductivity. Using new device designs, we were able to fabricate and measure stable quantum dots in this material system (PhysRevLett.).
- The stability of Majorana bound states depends crucially on the size of the topological gap. Using numerical simulations we found that, especially in 2D systems, straight wire geometries may suffer intrinsically from a small gap. We showed that a modification of the straight geometry into a meandering or “zigzag” geometry can improve the topological gap by an order of magnitude or more. These new device geometries are readily applicable to existing materials (PhysRevLett.).
- The properties of superconducting hybrid systems are routinely probed by conductance measurements. In an ideal system, the subgap conductance is expected to be symmetric with respect to the applied voltage, but experiments often find a distinct asymmetry. So far, all explanations of this asymmetry have relied on the existence of subgap quasiparticles that would be detrimental to future qubit performances. We showed theoretically that this asymmetry may also arise from reasons that would not be detrimental, and provided guidance on how to distinguish the different cases experimentally (SciPost).
Quantum computing architecture stack
Long-term goal:
To develop a scalable quantum computing control system stack that bridges the gap between quantum applications and quantum devices.
Highlights:
- We improved the OpenQL compiler – an evolving and mature quantum software platform for interfacing to quantum computing devices (arXiv). We enhanced its installation and availability and opened up the internal interfaces to better support integration of new components and adaptation to experimental environments. The OpenQL manual can be found here.
- To simulate larger algorithms (higher number of qubits) under realistic noise, with improved accuracy, we proposed a simulation approach that relies on approximating the density matrix evolution with a stochastic sum of unitary and measurement channels within a pure-sate simulation environment (Phys. Rev. A).
- We continued exploring efficient mapping techniques for the realization of quantum algorithms on resource-constrained quantum devices (DATE). We proposed the application of Design Space Exploration methodologies, for developing optimal mapping algorithms, but also to optimally architect full-stack quantum systems in the NISQ era (DCIS), and even to analyse the scalability of quantum processors based on a distributed multi-core approach (in collaboration with UPC) (arXiv).
Cryogenic electronics
Long-term goal:
To replace room-temperature electronics that control quantum processors with integrated cryogenic electronics, operating in close proximity to the qubits, in order to facilitate large-scale quantum computers.
Highlights:
- We demonstrated universal two-qubit control and quantum algorithms on silicon spin qubits controlled by a cryogenic controller implemented in Intel 22nm CMOS technology (Nature, in print, see arXiv). The cryo-CMOS controller (JSSC 2020) was awarded the ISSCC 2020 Jan van Vessem award for outstanding European paper.
- We integrated a switched-capacitor circuit with a quantum dot as a proof of concept for scalable DC biasing of quantum dot gates (Appl. Phys. Lett.)
- We showed the cryo-CMOS oscillator demonstrating state-of-the-art noise performance (ISSCC 2020).
- We advanced the understanding and modelling of cryo-CMOS devices, including both RF passives (JEDS 2020) and the matching of cryo-CMOS transistors (JEDS; and a second paper: JEDS).
- The building blocks for the cryogenic electrical readout of spin qubits has been presented at ISSCC 2021, including the readout of a double quantum dot with a cryo-CMOS RF receiver and the first high-speed cryo-CMOS analog-to-digital converter.
Theory for assessing the performance of quantum processors employing superconducting and spin qubits
Long-term goal:
To provide analyses and ideas towards implementing and assessing the performance of quantum error correction for superconducting and spin qubits
Highlights:
- Quantum annealing and variants of this method are commonly used to solve optimization problems on a quantum computer, using flux qubits as hardware platform. We showed that thermal and ground state properties of circuit-QED systems quite generally avoid the sign problem, and are hence amenable to classical Monte Carlo simulations (arXiv). Our analysis sheds light on the quantum computational advantages of these methods.
- We showed how qubit leakage can be detected with high overhead efficiency by processing syndrome information and using the distinguishability of a leaked state of the ancilla qubit (npj Quantum Information). Also, we presented novel hard-ware efficient scalable leakage reduction schemes (ArXiv).